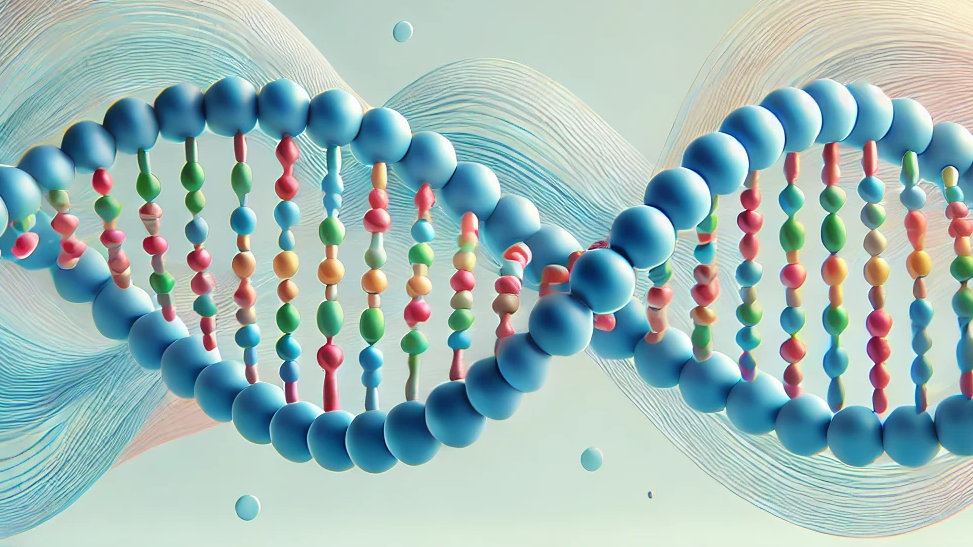
In recent years, gene editing technology has made significant progress, with CRISPR technology emerging as a revolutionary tool that has been widely applied to various gene manipulations. However, the core proteins in the CRISPR system, such as Cas9 and Cas12, while highly efficient and widely applicable, have large molecular sizes that limit their flexibility and effectiveness in certain applications. To address this issue, scientists have been exploring more compact and efficient gene editing tools. Recently, Nature Methods published a groundbreaking study from the University of Zurich on the TnpB system, which not only features a smaller size but also possesses powerful gene-editing capabilities, representing a new breakthrough in gene editing technology.
TnpB is a type of protein encoded by transposons, believed to be a precursor of the CRISPR system. Compared to CRISPR effector proteins like Cas9 and Cas12, TnpB has the advantage of a smaller size. Initially, the Deinococcus radiodurans (ISDra2) TnpB system was found to be usable for gene editing in mammalian cells. It utilizes an RNA molecule called ωRNA to guide the system, bind to target DNA, and perform cuts, functioning similarly to the guide RNA (gRNA) in the CRISPR system.
The research team optimized TnpB's codons and nuclear localization signals (NLS) to develop a new version called TnpBmax. This optimized version significantly enhanced gene-editing efficiency in mammalian cells, with an average improvement of 4.4 times.
The article identifies two key limitations to the TnpB system’s efficiency, and the researchers propose different solutions for each:
1: ωRNA Design
The design of ωRNA is crucial in determining editing efficiency. The research team established an experimental library containing 11,188 target sites to thoroughly analyze the design principles of ωRNA. They found that TnpBmax prefers sequences rich in purines (A and G), particularly in the first 12 bases of the ωRNA, where mismatches are not tolerated. This design ensures that ωRNA has high target specificity, reducing off-target effects and enhancing the safety of gene editing.
2: TnpB's Dependence on TAMs
TAM (target-adjacent motif) dependency limits the system's targeting range. To overcome this, the research team introduced mutations at the K76 site of TnpB, generating several TnpB variants capable of recognizing atypical TAM sequences. Among them, TnpBmaxK76A showed high recognition for the 5'-TTtAT TAM sequence. By introducing this mutation, the researchers expanded TnpB's targeting range from the original 5'-TTGAT to 5'-TYKAT, which occurs four times more frequently in the genome, greatly enhancing TnpB's application flexibility.
The compact size of TnpB allows both TnpB and ωRNA to be loaded into AAV (adeno-associated virus) vectors, enabling in vivo gene editing. Through experiments on mice, the researchers found that using AAV to deliver TnpBmax achieved up to 75.3% gene editing efficiency, particularly in the liver and brain of mice, demonstrating excellent results. This shows that the TnpB system not only performs well in in vitro experiments but also has great potential for in vivo applications.
As a compact gene-editing tool, the TnpB system offers advantages such as high efficiency, strong specificity, and a wide targeting range, especially promising for in vivo gene-editing applications. Notably, to simplify the design of ωRNA, the research team developed a deep learning model called TEEP, providing a more precise tool for TnpB design and application, significantly reducing experimental costs and time.
This study marks another important breakthrough in gene editing technology, laying a solid foundation for future applications in gene therapy, disease research, and other fields. Feel free to read the original paper via the link below.
原文阅读:https://doi.org/10.1038/s41592-024-02418-z